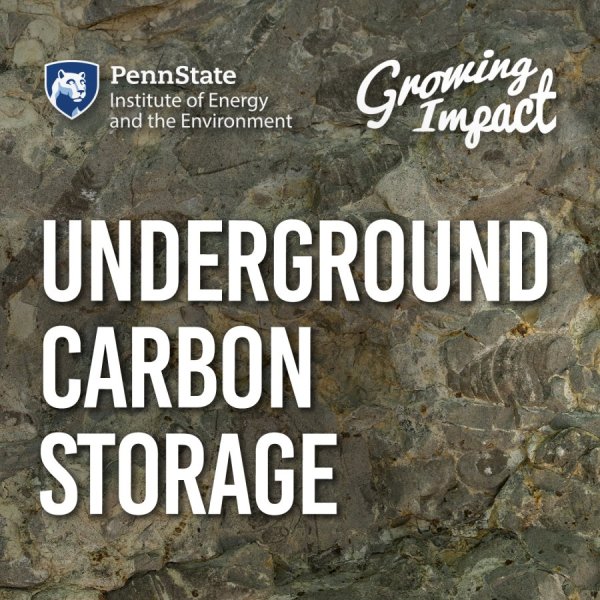
39-minute listen/watch | 28-minute read | 1-minute teaser
Storing carbon dioxide underground could offset the record amounts of CO2 that humans emit each year. If this technology is successful, it could be an answer to climate change impacts, such as rising temperatures and acidification of the ocean. However, the process is complex and costly. A team of researchers is exploring one way liquid CO2 could be injected into rock formations, which may efficiently convert the molecule into a solid.
Transcript
Anne Menefee
There's a lot of operational experience with the same technologies that you would use to do dedicated carbon capture and storage, but I think a lot of the current barriers to it, to deploying it, as, you know, a large-scale climate change mitigation strategy largely come down to risk, as well as cost and logistics.
Host
Welcome to Growing Impact, a podcast by the Institute of Energy and the Environment at Penn State. Each month, Growing Impact explores the projects of Penn State researchers who are solving some of the world's most challenging energy and environmental issues. Each project has been funded through a seed grant program that's facilitated through IEE. I'm your host, Kevin Sliman.
Human activities are releasing more carbon dioxide into the atmosphere than natural processes can remove, causing a steady rise in its concentration. In fact, the global average reached a record high in 2023, which is 50% higher than the pre-Industrial Revolution levels. These high levels of carbon dioxide are increasing its greenhouse effect and warming our planet. One way to address the situation is to capture and store CO2 underground. On this episode of Growing Impact, we discuss the process of injecting CO2 underground, the risks and rewards associated with it, and how two Penn State researchers are helping improve our understanding of a complex underground process that may be a key factor in climate change mitigation.
So welcome, both of you, to Growing Impact. I really appreciate you taking time to talk with me about your research. And the first thing I'd like you to do, could you each introduce yourselves? So can you tell me your name, your title, and give me a brief background of your research?
Yashar Mehmani
Thanks, Kevin. It's good to be here. My name is Yashar Mehmani. I'm a faculty in the Energy and Mineral Engineering Department at Penn State University. My research, briefly, focuses on porous materials, understanding how fluids flow through them, how reactions alter their internal structure, and how mechanical forces may cause them to deform or break. And I study these processes both from a computational perspective and try to mimic how these processes are occurring at the microscopic scale within the nooks and crannies of the material and try to relate those tiny processes to some observables and some measurables at the human scale.
Anne Menefee
So my name is Anne Menefee. I'm an assistant professor in the same department, so the Department of Energy and Mineral Engineering here at Penn State. I'm an environmental engineer by training. And my research broadly is motivated by climate change mitigation. So currently I do a mix of experimental work, reactive transport modeling, and life-cycle analysis related to technologies like subsurface carbon sequestration, carbon mineralization, and emerging technologies to support decarbonization like hydrogen storage and geothermal energy.
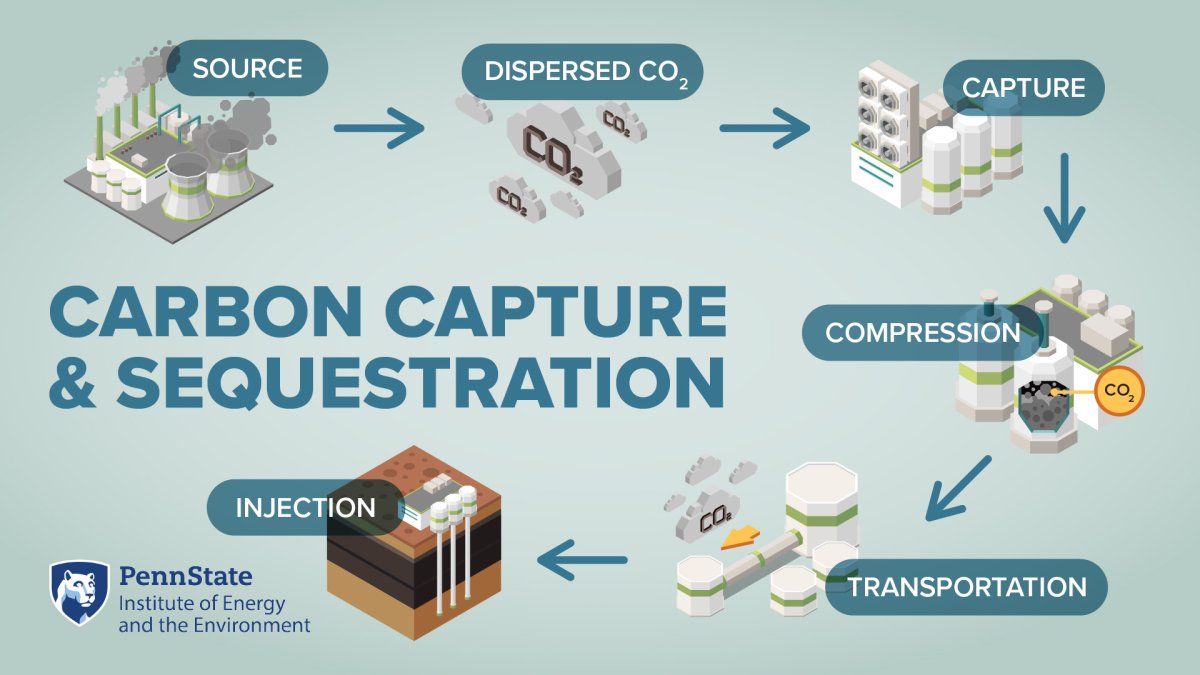
Host
Your work involves the formation and dissolution of minerals. Can you explain how these functions occur? And second, can you provide some examples that people may be familiar with?
Anne Menefee
Yeah. So I guess the... probably the easiest way to explain it is that whenever you inject or extract a fluid in the subsurface, you're going to be changing the chemistry of that environment in some ways. Those changes can cause minerals that are in a rock formation to dissolve, and it could cause new minerals to precipitate or fall out of solution.
Probably the simplest example I can give is that if you know you dissolve a solid block of salt in water, it's going to dissociate into its ionic constituent—so that would be, you know, sodium and chloride for table salt. And then precipitation is essentially the reverse of that. So if you have a solution that is overly saturated with the ions that can form a precipitate, that solid phase can fall out of solution essentially. So I guess a simple example of this would be, you know, we live in a region with very hard water. So, if you've ever seen, you know, scale build up on pipes or faucets, that's probably a carbonate mineral that has fallen out of a supersaturated solution.
Yashar Mehmani
So if you want to think about dissolution, I think, even though this is a very different example I'm going to give is a different process, but the ultimate result is the same. So, for example, if you just imagine pouring hot water on ice, it starts to dissolve, right? So it's a process of solid subtraction. So the solution is when you start from a solid and then the solid gets less, it sort of melts away in the fluid that it’s swimming in. And precipitation is the opposite. It’s a process of solid addition.
Childhood example for me was, you know, if you dissolve a lot of sugar in water, at a high temperature, right? So to get it to boil and just dump a bunch of sugar in it and then slowly start to cool it and put little strings inside, you'll see, sugar crystals forming, and that precipitation is sugar is coming out of the solution. And you're adding solid to these little strings.
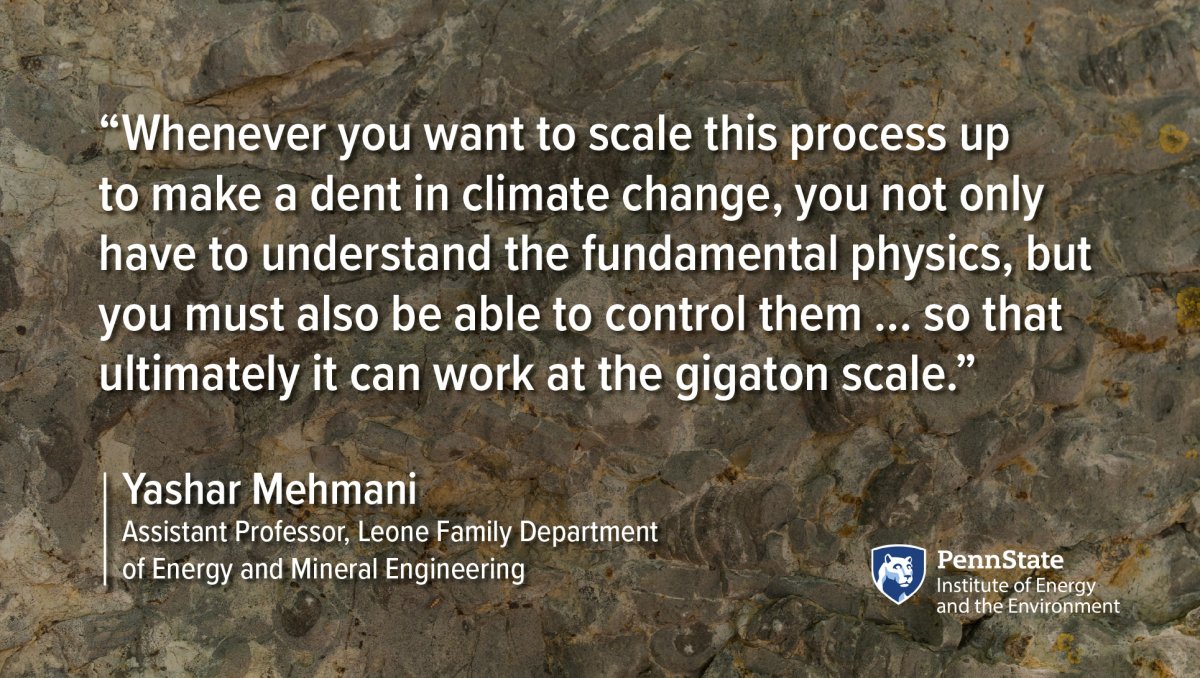
Host
I was thinking that exactly. Actually, I was like, I remember making, like, little rock candies out of sugar water. Perfect. Excellent. Well, thanks so much. Great explanations.
Yashar Mehmani
It’s a Persian delicacy. That's how we drink tea.
Host
So then you use the sugar from the strings and use that...
Yashar Mehmani
Yeah. You put, like, a little piece of wooden stick and, in that supersaturated, sugar-saturated solution. And then you get a bunch of crystals. Usually it's infused with saffron. And then once you have that sugar candy, you dunk it in your tea. Haven't had any in years. But yeah.
Host
That sounds like a delightful way to do it. So why is it important to understand how minerals form and dissolve?
Anne Menefee
It's super important to a lot of environmental systems in particular. You know, I have a background in environmental engineering, as I mentioned. And so it's very relevant in water treatment, wastewater treatment processes. But I guess, you know, more relevantly to the work that Yashar and I are doing right now, it's super important for subsurface engineering and, and especially in the energy sector, right? Anytime you're extracting a resource from the subsurface or injecting something like carbon dioxide, which is a weak acid that both, you know, dissolves into essentially the groundwater and, and can react with the surrounding rock. Or if you're relying on a rock formation to store some sort of energy or waste product, in any of those systems, you need to ensure that the fluid itself is not going to react with the surrounding rock in a way that compromises the integrity of that formation, or reduces the performance of whatever, you know, engineering goal you're trying to achieve.
And different rocks have different, I guess, sensitivities to different fluids, right? Some rock formations like basalt, which we can get into a bit because that's, you know, very relevant to the research we're doing, are quite reactive with acidic fluids. So CO2, for instance, is a weak acid. It can spark a lot of dissolution of certain minerals. But other formations like sandstone and other sedimentary rocks are quite inert and may not experience much alteration or deformation due to chemical reaction. So understanding how minerals are, you know, to get back to your last question, how minerals are dissolving and precipitating in these environments is crucial to being able to predict and even control fluid transport through the subsurface for applications like carbon storage, geothermal energy, or understanding things like groundwater flow and the fate of contaminants underground.
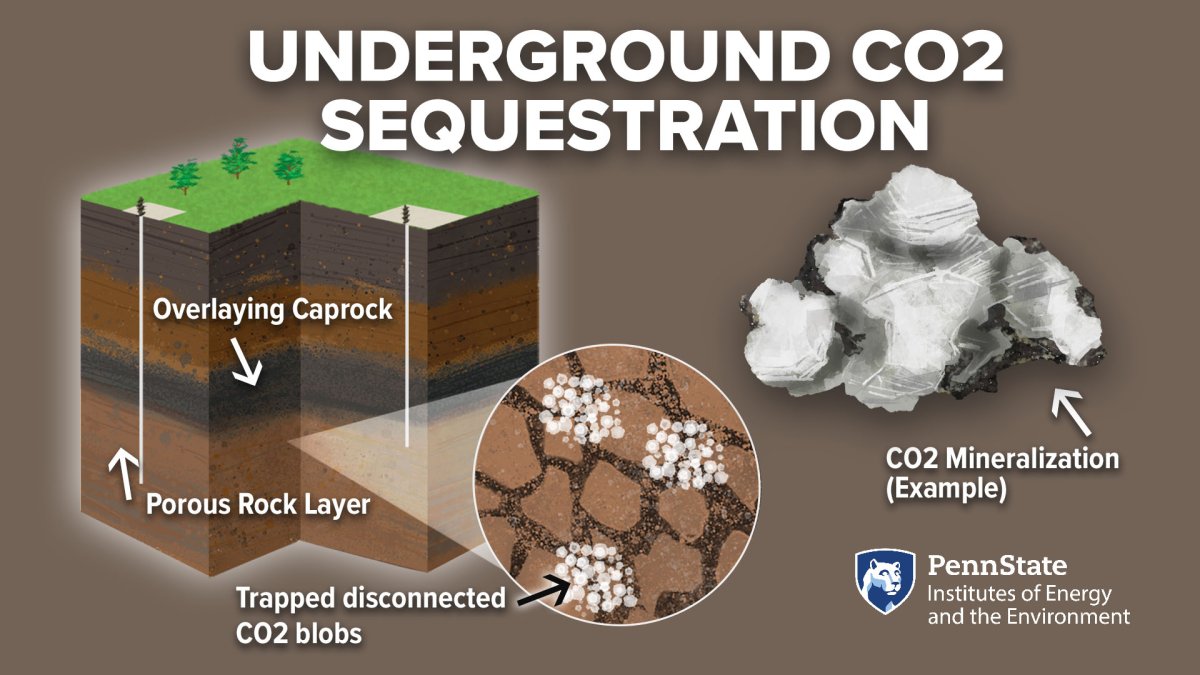
Yashar Mehmani
One thing you can do is you can look at the current state of rocks under a microscope. And if you understand how the minerals came to be, and you know, how they could dissolve and how they could form, you could infer the history of the earth and the climate history of the Earth. That's not what I do. There are a ton of people that do this kind of stuff. So you can sort of like look at a crime scene and sort of deduce what happened before that.
In the context--so this involves a very long time scales--in the context of the human timescales for engineering, which is at least my expertise and Anne’s expertise, I think of dissolution and precipitation, the importance of it as ways of if you want to get rid of, dispose of a compound, you just don't want it, it's a waste. You probably want to lock it. You want to precipitate it. You want to dissolve it, and then you want it to sort of precipitate it out from that, from that solution. Because once it's precipitated, it's immobile. It's a piece of, you know, hard rock sugar, and it can't go anywhere.
Whereas if you want a commodity that's already locked in and you want to unlock it, you want to dissolve it, right? And this could be... this could be rare earth minerals. Right? So if you want to, that are used in electronics, maybe you want to leach them. Maybe you want to acidify, inject some acid and try to dissolve the rock or dissolve the compounds that you really care about and then extract them out of the rock. I'm sure people mining are thinking about this. And sometimes you accidentally end up unlocking things that you don't want to unlock, like contaminants, like strontium or chromium and a whole bunch of other ions, heavy metals that you're injecting something to either lock or unlock something, but accidentally unlock something that could contaminate groundwater and end up in your body.
So this is why it's important to understand these locking and unlocking mechanisms. So in my work, I'm interested in all of these locking-unlocking mechanisms that are occurring within a complex geometry that constitutes the empty space, the void space of a porous medium. Right? So imagine a porous medium, you have a cube, and there are a bunch of holes in it. The holes are connected and the solid contains a whole bunch of minerals. It's heterogeneous, right? It may contain calcite, may contain dolomite, it may contain quartz, all sorts of things. And the void space are access points for fluids. Fluids are flowing through them.
They're carrying a whole bunch of solutes and things that you have dissolved, things that you've accidentally moved through, and they're all passing by these minerals that are sitting, sitting around it. And so in that context, if you precipitate or dissolve, you're changing the geometry of, of this pore space. You're changing the character and the properties of this thing. You're changing its mechanical properties, you're changing its hydraulic properties. And so, so as these reactions are occurring, and you're locking some minerals and unlocking others, your porous medium evolves in every way. Right? And so this has implications for engineering these things at large scale.

Host
Do either of you work in the area? And I think, Yashar, you mentioned that you did, but I'll clarify. In identifying ways that it will change, are you utilizing models and other ways to predict or to foresee how potentially that would change from an engineering perspective?
Yashar Mehmani
Potentially, yes. Yeah, that's generally what we do in my group. We develop models to understand how porous materials evolve due to various forcings onto the material. And in this case, that is a key question that's driving the collaboration between me and Anne.
Anne Menefee
I'd say, my research is focused a lot more on fractured materials as opposed to porous. But there's, you know, a lot of synergy. And in any sort of subsurface, you know, porous reservoir, part of that, you know, “pore volume,” quote unquote, is, is in the form of fractures. Right? Fractures are just a different kind of, you know, void space in the subsurface. And, and there are certain systems in which we want to prevent fractures. Right? If you're trying to store CO2 underground, you probably want to have a cap rock on top of that reservoir that is not fractured because you don't want the CO2 to migrate out. And then there's systems in which we might want to create fractures. Right?
A good example of this is, you know, the shale gas industry there, stimulating the reservoir via hydraulic fracturing to generate enough permeability in the rock for natural gas, for instance, to flow out of the reservoir. Another example would be, stimulating reservoirs for geothermal energy production. Right? If the reservoir itself doesn't have enough connected pore space, as Yashar was just talking about, for fluids to flow, you might have to induce fractures. So I think, you know, understanding how these sorts of reactions impact flow in porous and fractured materials is super important for a lot of subsurface engineering and just in general environmental applications.
Host
Can you discuss porous materials and the role they play in your work? And you have already, I mean, you've given some indication on it. Do you have anything you think you would like to go further into the discussion about porous materials?
Yashar Mehmani
When I started my PhD, the only porous materials that I ever... that I knew about and I focused on were rocks. I thought that that was pretty much it. Right? Sedimentary rocks that were formed through the deposition of a whole bunch of stuff that’s constantly depositing at the bottom of oceans and lakes. And so I wasn't thinking out beyond that. But then over time, I realized there's hardly any... very few examples that are around us that are not porous.
So, for example, the prime example is that Earth itself is porous. Right? So not just that the medium-depth stuff that I was working on during my Ph.D., but the top soil, the soil is porous. The roots of trees are porous. If you cut the stem of a tree and look at the cross-section, that is porous, if you wander in your building the plaster, I don't have any plaster, but you know the plaster on your walls, Kevin. That's, that's porous. And the bricks or the concrete that's sitting behind that is porous, the wooden doors and the wooden furniture that you have, those are porous. That's why you have water damage. And and so that's just talking about the Earth. Right? And if you if you forget about rocky materials or forget about... And this , by the way, applies to other planets. Other planets are made of the same dirt, right, excluding stars. And so in, in other applications, like our body is porous, our flesh is porous and the fluid is...
And so it’s a form of a porous material and, you know, we have blood and plasma and all sorts of nutrients that are dissolved the same way that we discussed. And there are a ton of reactions that are happening, and our bones are porous. So evolution has beautifully shaped us so that we don't weigh too much. So we conserve energy, but at the same time we're strong so that we don't we're not very flimsy.
So our bones have a very beautiful internal structure that has been, so to speak, optimized over millennia. And, and yeah, and the list goes on and on and on. And you know, in energy applications you find porous components in all sorts of things like gas diffusion layers for fuel cells or battery electrodes and so on and so forth.
So I've come to know that it's one of those things that people don't know about. My family members ask me what do you work on, what I work on, and I say porous materials. They’re like what? And it's everywhere.
Host
So in your project, you're looking to develop a model that will figure out the best ways to assess and optimize the storage or transformation of carbon dioxide in underground areas. Can you discuss a little bit about the model and what it will help you understand and hopefully ultimately do?
Anne Menefee
So to kind of dig back in a little bit to this specific research. The field application of this work is the underground storage of CO2 in basalts and other mafic rocks, which supports carbon mineralization. So taking a step back, you know, we've kind of thrown out some, some different geologic terms here. There are three basic rock types, right? And mafic rocks are considered igneous rocks. So think, you know, rocks that form from lava like you would see in Hawaii or Iceland, the Pacific Northwest, they tend to be very dark in color and essentially basalts and other mafic rocks like this are very reactive with CO2, which is I, I think I mentioned before, CO2 is a weak acid.
And so if you inject CO2 into one of these rock types, that sparks a series of geochemical reactions through which the CO2 is actually converted into a solid carbonate mineral. So, kind of like Yashar was talking about and so eloquently worded this idea of locking versus unlocking things in different materials, you're essentially locking the CO2 away where it's permanently going to be stored over extended geologic time frames, as opposed to held in some sort of mobile phase, kind of where it has potential to flow underground. So this has a huge advantage in terms of CO2 storage security, which has been, one of the leading, I would say, barriers to increased deployment of carbon sequestration systems because you're essentially solidifying it underground.
This concept has been demonstrated to some extent at lab and at pilot scale. But, it's certainly not a mature technology. And I think there's a lot that we still don't have, you know, satisfying answers for, like, where is the basalt dissolving versus where are these carbonate minerals precipitating, throughout a given reservoir? And I think, you know, one of the long-term goals of this research is going to be to kind of help constrain where these reactions are occurring, which is critical to estimating storage capacities and I guess the last thing I'll say on this is that this kind of idea of mineralization has, has often, I think especially when it was first conceptualized, it was cast as this trade off between CO2 containment assurance that you get from solidifying the CO2 and reservoir performance. And by that I mean that if you, you know, solidify or mineralize something underground, you're going to be filling up pore space and reducing the permeability of the reservoir or essentially limiting your ability to continue injecting more CO2 into that reservoir.
So, there's kind of this idea that, that there's a bit of a tradeoff between, you know, solidifying the CO2, but also preserving permeability so that you can continue injecting it and maximizing how much CO2 you can mineralize within a given system. So understanding kind of how these reactions distribute throughout a given pore space is really critical to kind of balancing these two end points.
Yashar Mehmani
If you zoom out and you say, well, what do you want out of this technology? What do you want to know? It’s “how much can you store?” and “how fast?” Right? And so. And there are kind of, as Anne was pointing out, there are kind of on two ends of a seesaw. And so if you store a really fast, if the reactions are really, really fast, you're going to clog up the pore space. So you have a well and then you're injecting all this stuff into a formation. Imagine a pancake and they're sort of spreading outward. Right? If the reaction happens really fast, they're going to clog up the area around the, around the well. And now suddenly you can't inject anymore because you've plugged it up. Right? And so you want, you want to be slow enough so that it travels out.
And, and generally this is not an issue because these reactions tend to be slow anyways. But you also want it not to sit there for very long because the longer it sits, the risk of it breaching and leaking also increases. So you want it to mineralize quick, but not too quick. And also this beautifully ties in with what Anne was describing in terms of like the extent of storage. Like how at the end, once you're done with this well you move away, how much CO2 did you actually sequester, turn into rock? And this question depends on, well, how far were you able to get the CO2 out and how much of it actually converted, because there was a bunch of rate-limiting steps as Anne was pointing out, you need these cations. You need these. You can't do this with every rock. These are special rocks, mafic rocks that that contain divalent cations like magnesium and calcium, that that sort of combined with the CO2 to form these rocks. Right? So and so will you have enough cations in this particular rock? Are you going to run out? Are there other factors that will prevent the combination?And so yeah, those are the, I would say, some of the key questions.
Host
As you're working through this. As you're researching and doing experiments, how easy or difficult is it to replicate this in either a lab or in some other fashion, whether it's a model or otherwise, and then successfully get it to, you know, operate in that way, in real life, you know, and in a real situation?
Anne Menefee
Yeah. Yeah, I think definitely. I think we can, I guess the first thing I'll say is, you know, I don't operate like a CO2 injection facility at scale, but that's exactly what we try to do. Right? Like to understand these processes as they would be carried out in the field. And so, you know, to your question, it's definitely, you know, I've done quite a lot of experimental work on CO2 mineralization in basalt. And I guess I'd say it's certainly not trivial to replicate, subsurface temperature and pressure conditions during reactive flow of fluids through, you know, a fracture network. I've done quite a bit of experimental work as well, where I was actively fracturing samples to simulate certain conditions in certain environments while, you know, subjecting them to this reactive flow and then trying to obtain the right experimental measurements, trying to capture live images of these samples.
So there's definitely quite a bit of complexity in trying to just to study and understand and constrain these reactions under representative subsurface conditions. But I think, you know, that's part of what makes research exciting, right? It's doing things that people haven't done before and being able to add new contributions to science and to our understanding of these systems.
And I actually have seen in, you know, a lot of our experimental work and I'm sure, Yashar can speak to this as well, a bit more on the modeling side, but we actually have been able to, you know, kind of observe some of the phenomenon that have been observed at larger, like pilot scales with CO2 injection into basalt and be able to dig into things a little bit deeper and explain why they might be seeing certain things. For instance, why maybe they aren’t seeing a loss of permeability despite the fact that their reservoir models tell them that they should be, and we can look at things experimentally and based on the distribution of how these reactions are occurring throughout a fracture network or a pore network we can start to provide some answers to that.
Yashar Mehmani
Yeah, that's, Anne answered it beautifully. I mean, the question that you asked Kevin, that's an ever-present canonical problem of geosciences. Right? So, or geo engineering. How do you connect what you do or see in a lab? And Anne has a lot more experience in the lab doing with these systems than... I have I haven't done any lab experiments related to this, but relating what's what you see there or even in the models earlier I said, you know, I do, I look at these tiny scales and I model the processes at these tiny scales. But the, you know, the reservoir is several kilometers wide. So doing that connection is not trivial at all. And it involves piecing together various observations at various scales, you know, data collected at, at the field site, larger scales at different time scales. Maybe you want to look at geological analogs. You have all sorts of data, and all sorts of modeling tools or experimental tools that you could you you should utilize if you have it, to sort of constrain the problem as much as possible, because there is a lot of uncertainty with it. But that doesn't mean that you can't constrain it. You can.
Anne Menefee
Right. And I guess I would just add one, you know, quick piggyback to that. Both piggyback to Yashar’s point and kind of, you know, to tie a bow on, on your original question. I think one of the unique challenges to geoscience, like Yashar was mentioning and, and to studying subsurface processes is that, you know, we can't stick a camera down there and see exactly what's going on.
Right? And so even in field scale, you know, testing and operations, we're relying on a lot of, you know, geophysical or geochemical measurements and then making inferences about what's actually happening in that subsurface pore space. And so it can be a challenging thing to try to, to study and try to connect between, you know, experiments and models and, and reservoir scales because you don't have that, you know, kind of ground truth data of we have observed exactly what is going on within this pore network in, you know, an actual reservoir.
Host
And the other thing I could think of. And I'm sure this is already on your mind. Is that it's a system that is dynamic. It's changing. It's not like the Earth is in one position and will never move or change or, you know, it's. There's chemical reactions and movement and yada, yada. So that's a whole other level of variables that you get to contend with.
Yashar Mehmani
Yeah. And you can think of it this way, that even if the Earth were in equilibrium, which it's not. But even if a piece of, a chunk of reservoir is perfectly happy the way it is, by the virtue of the fact that you're injecting fluids and withdrawing fluids in it, you now put it in a state of disequilibrium. And so it wants to get to a new state of happiness. Right? So it has to change to become happy because it's not. You made it unhappy.
Host
You alluded to this at some point about CO2 storage in relationship to climate and climate change. Can we discuss the storage of CO2 when it comes to carbon capture and climate? And what do we know? And what are we still figuring out?
Anne Menefee
Well, the good news is that we actually know a lot, I would say. The first geologic CO2 storage project actually predates me. There's a storage site in Norway that's been injecting CO2 since the early 90s. And, you know, the oil and gas industry also has a lot of experience injecting CO2 underground in the U.S. They've been injecting CO2 for enhanced oil recovery since the 1970s here in the US and in western Texas. And so I think there's a lot of operational experience with the same technologies that you would use to do dedicated carbon capture and storage.
But I think a lot of the current barriers to it, to deploying it, as, you know, a large-scale climate change mitigation strategy largely come down to risk. You know, there's there's still some geologic risk, which we've kind of been alluding to throughout this podcast, as well as cost and logistics.
And on the cost side, the recent amendments to the in the passing of the Inflation Reduction Act have increased available tax credits for capture and storage of CO2. Right? There's different credit rates available depending on where you're capturing that CO2 from. If you're getting it from, you know, a point source like a smokestack versus if you're removing it directly from the atmosphere, there's a bit more money available for, for CO2 removal from the air.
But we're seeing a lot more activity in companies applying for EPA permits that are required to be able to inject CO2 since those credits were increased. So the size of the prize is growing. But I would say there's still a challenge with, you know, the amount of CapEx, amount of infrastructure that's required to support large scale capture, transport, and storage operations. And right now we don't have we don't really have dedicated financing instruments in place to support that on a national scale. I would say, though, and, you know, in terms of risk, kind of to, to circle back to, to our research focus, the specific angle of carbon sequestration that Yashar and I are focused on here, mineralization, which we've been talking about actually, you know, circumvents some of the geologic risk and some of the concerns related to storage security or leakage of CO2 or I guess, you know, some prefer to call it unwanted migration of CO2 out of the reservoir, because if you're working in a reservoir that's capable of mineralization, like we've said, you're converting the CO2 into a solid phase and essentially immobilizing it underground, right?
So this is kind of a natural means of stabilizing the CO2. But like we've said, it's really important to understand, you know, where these reactions are occurring. The timescales over which they occur and the extent to which they occur, so that we can truly understand, you know, the risk as it relates to how much CO2 we may be injecting.
Yashar Mehmani
I'll add a little bit of maybe background to what Anne was talking about. There are various ways of getting rid of CO2 by injecting it underground. There are various mechanisms through which you, quote, unquote, trap the CO2. One of the earlier ways, the one in the project in Norway that Anne was talking about is through a process where you inject CO2, not within a particular mineralogically special reservoir, right? The reservoir itself is kind of boring. It just has a lot of pore space and it has a nice caprock, so it's sealed. And so you inject it and because it's so deep, the CO2 becomes supercritical, which is, which is a not liquid, not gas-like state. And so it sort of, pushes back against the water that's sitting in the, in the reservoir.
And then it's sort of like, like olive oil and water, it sort of like beads up and into droplets and so on. And the droplets are good or, or perceived to be good. And so it's, it's a good thing to break up the CO2 into these little isolated droplets in your, in your formation, because the thinking is that then they wouldn't escape. They're trapped. That's called residual trapping. But over time, the CO2 dissolves in the water, right? It dissolves, but not super fast, but it does dissolve. And then when it dissolves, the water gets heavier, it intensifies, the density goes up, and then sort of sinks to the bottom. And that's also a good thing. That's called solubility trapping.
But in the process you're sort of acidifying the fluid because when you carbonate water, just like you have open a can of soda. It's acidic. Right? So you might dissolve or, you know, unlock some, some stuff. So, these are so those are called like that. That's the normal, usual CO2 sequestration mechanisms, solubility trapping and residual trapping and structural trapping.
The project that Anne and I are working on is on one subset of this that's the most stable, called mineral trapping. So when you convert CO2 into this mineral, and if you if you were to do this in the, in the normal boring kind of formation, it would take a very, very long time to get there. The nice thing about these special formations, these mafic formations that have these cations that we talked about, is that this process is presumably really fast. Right? And it's also once you convert it to CO2, you don't have to worry about leakage. Whereas in this other way of sequestering CO2, the CO2 is still there, right? It's liquid. So, will it mobilize if there was a little earthquake or if things move? If there is background flow, you know, there are questions related to...
And then the CO2 also rises volume, sort of sits underneath caprock and it may eat away at it. So there is a lot of fundamental science that is known about both all of these things. But whenever you want to scale this process up, to say, make a dent in climate change globally, that's when you have to have more constrained answers.
You have to have more understanding of the fundamental physics. You have to not only understand them, but be able to control them. And I'm not sure we're there yet. Right? So this this is why, you know, projects like this, that collaborations like this between Ann and I are helping to get that reliability so that the ultimately you can scale this on, on all sorts of reservoirs and the gigaton scale.
Host
What are some of the next steps for this project?
Yashar Mehmani
So if you understand a process, right? If you this holds for anything really in science... if you understand a process you're you're able to predict what's going to happen. Right? So you can build a model, you can predict, okay, if you give me these situations, these set of inputs or conditions. This is this is what the outcome is going to be. And so in this context that we've been we've been chatting about, building a model that kind of you can reliably predict some of these processes would be... is something that we've initiated. And what, you know, what's, a tangible outcome of this project hopefully would be. But then, you know, any time you build a model, you have to constrain it to experiments too. Otherwise it can produce outcomes that are completely unphysical.
So there are, you know, and Anne is doing a lot of experiments to sort of like help constrain the model in that direction. And our hope is that once that's done, we could once we have a model that you can rely on, then you can start asking questions about optimization. Okay. So what top what knobs do I need to turn in which direction so that I can maximize extent of carbonation, for example, or, or minimize rate of carbonation. So that's, that's sort of where we're headed.
Anne Menefee
Yeah. I think that was perfect. And I guess just to add, in terms of, you know, applications, I mentioned before, we we're seeing kind of a flurry of activity of facilities, different companies applying for permits to inject CO2 and so the to be able to store CO2 underground you have to obtain a permit. And these are regulated by the Environmental Protection Agency in the US through the underground injection control program.
There's a separate class of wells for CO2 injection. And that’s separate from, you know, existing classes of wells for injecting wastewater and injecting different hazardous waste streams and injecting CO2 for enhanced oil recovery, as I mentioned before. And, and any other, you know, reason, we need to inject a material underground. And part of that application process is a pretty thorough characterization of the target storage reservoir, as well as demonstration that you've predicted with a pretty high degree of certainty exactly where the CO2 plume is going to go, how it's going to transform, you know, how much of it is going to dissolve into the formation brine, how much of it could be held by those different trapping mechanisms that that Yashar detailed, you know, structural versus solubility versus residual trapping and, you know, in the case of basalt formations, predicting, you know, how much of it could be immobilized within a given time frame, because that has significant implications for, for storage security and storage permanence. Right?
And so, I think long term, developing better predictive tools and capabilities to really understand what's going to happen before we start injecting CO2 has a lot of really important implications for, for regulators and for companies and facilities that are trying to do this at an industrial scale.
Host
And success. I know the seed grant is a place where you're gathering data and building a foundation to move forward. So whether you want to speak to maybe what success might look like at the end of a seed grant project, or maybe, as you would move on to the next steps, something that you would be looking for or what you would consider a success.
Yashar Mehmani
In my view, success would be if we... The first order success would be if we actually understand at a fundamental level what some of these processes do in the short run and in the long run. Where do the precipitates occur? And do we have enough trust in the models to sort of rely on doing that optimization? So, so understanding, really understanding this is I would say, and we're not going to solve it on our own. It's a very big problem, and a lot of people are working on it. But if we can contribute towards that, I think that's that in my view. And I'm sure in Anne’s view would be success. On the other end, hopefully the seed grant would snowball into larger grants, and then we could get students that are trained in this, in this area.
And that's a good thing, not just for science, but also for these students, you know, graduating and, assuming jobs in society that, that sort of propagate this and make this happen. Right? And there's, there's not enough people to, to, to make it happen right now and that, that so you need training and this we, we talked about how complex this problem is. And so you need people that know the complexity and so that they can make good judgment, when they are in industries or nonprofits or in companies, that would be another longer term success.
Anne Menefee
I think the goals are both to improve our understanding of this. To come up with very well constrained evidence to support, you know, a lot of our our hypotheses about how these reactions occur, where they occur, and how that's going to impact both things that we care about, like CO2 and activity and storage capacity. And also being able to instill some of this into the next generation and make carbon sequestration, you know, happen and make it contribute towards making it feasible and, you know, self-sustaining in the market and making it safe and and effective for long term permanent carbon removal as a climate change mitigation technology.
Host
Thank you both for being on Growing Impact. I really appreciate your time.
Yashar Mehmani
Thanks for having us. This was great.
Anne Menefee
Yeah, definitely. Thank you so much, Kevin. Really appreciate it.
Host
This has been season four, episode ten of Growing Impact. Thanks again to Yashar Mehmani and Anne Menefee for speaking to me about their project. To watch a video version of this episode and to learn more about the research team. Visit iee.psu.edu/podcast. Once you're there, you'll find previous episodes, transcripts, related graphics, and so much more.
Our creative director is Chris Komlenic, with graphic design and video production by Brenna Buck, and promotional and social media support by Tori Indivero. Join us again next month as we continue our exploration of Penn State research and its growing impact. Thanks for listening.