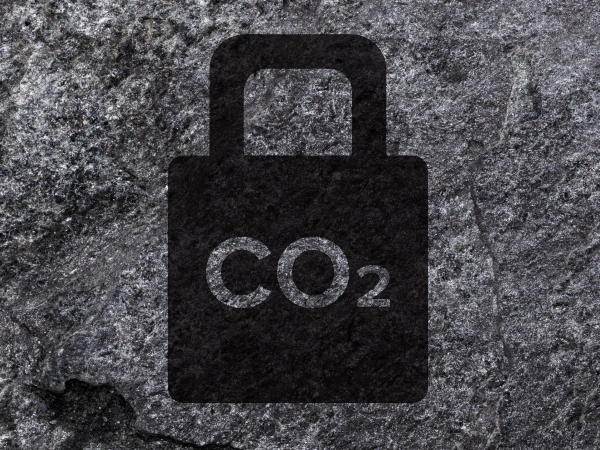
Addressing the incontrovertible risks of climate change requires deep decarbonization. In addition to green measures like upscaling renewables and improving energy efficiency, there is broad scientific consensus that large-scale carbon capture and sequestration (CCS) remains critical to limiting global temperature rise below 2°C. CCS involves capturing CO2 (from a point source or directly from the air); compressing and transporting it via a pipeline; and storing it deep underground, or utilizing it as a feedstock or agent in another industrial process.
The idea of CCS has been around for decades, and the first commercial project (where CO2 is captured and injected at the Sleipner gas field, offshore Norway) has been in operation since 1991. But until recently, CCS development has been limited by a lack of incentives or regulations. With recent increases in available tax credits (carbon credits) for CCS, there has been a surge in companies applying for Class VI permits, which are required through the EPA’s Underground Injection Control program to begin any CCS project.
In conventional CCS, CO2 is captured from a point source like a power plant, manufacturer, or other industrial facility. CO2 is typically collected by dissolving it into a liquid (absorption) or capturing CO2 molecules that adhere to the surface of a solid material (adsorption). Point source CO2 capture is a carbon abatement technology that prevents CO2 from entering the atmosphere.
CO2 can also be captured directly from the atmosphere using direct air capture (DAC) units, which cycle air through air-liquid contactors and use similar technologies as point source capture to concentrate ambient CO2. DAC is a carbon removal technology that can remove legacy CO2.
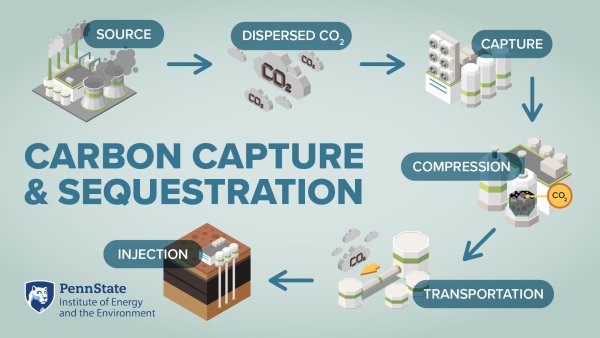
Once CO2 is concentrated from a source, it is compressed and transported via pipelines to an end use (i.e., a form of CO2 utilization or storage). There are many industrial uses for captured CO2, including feedstocks in the food/beverage industry, pharmaceuticals, and CO2-enhanced oil recovery. However, the sink for captured CO2 that is most capable of achieving gigaton-scale emission reductions is dedicated CO2 sequestration or storage. Most of our capacity to store CO2 on such scales lies underground. CO2 injection and long-term storage in the subsurface is referred to as geologic carbon storage or GCS. In GCS, CO2 is compressed at the surface and injected into a storage reservoir as a supercritical fluid. Prospective storage reservoirs undergo extensive geologic characterization to ensure that there is sufficient capacity to safely inject and retain large volumes of CO2, along with a secure overlying caprock to prevent the CO2 from escaping out of the storage reservoir.
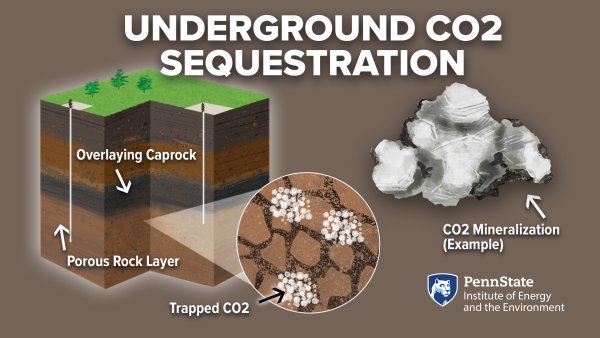
GCS has conventionally targeted sedimentary geologic formations, which generally have high permeability and porosity to support CO2 injection and retention, and are geographically ubiquitous in the continental U.S. Because most oil and gas are extracted from sedimentary basins, the petroleum industry also has decades of experience engineering these formations that can be translated to CO2 injection. When stored in a sedimentary basin, CO2 is initially trapped beneath an impermeable overlying caprock (structural trapping). Over time, the CO2 plume will start to migrate and dissolve into pre-existing brines within the reservoir. GCS in sedimentary basins is currently the most technologically mature form of GCS and has been demonstrated in various pilot projects, but there are still questions surrounding risk and storage security, particularly if deployed at industrial scales.
Alternatively, CO2 can be injected into basalts or mafic formations, which are igneous rocks that form from the cooling of lava. These formations are capable of mineralization, or mineral trapping. When CO2 is injected into the mafic rock, a series of chemical reactions between the injected CO2 and the surrounding rock convert the CO2 into solid mineral phases. This process is referred to as carbon mineralization, or mineral carbonation.
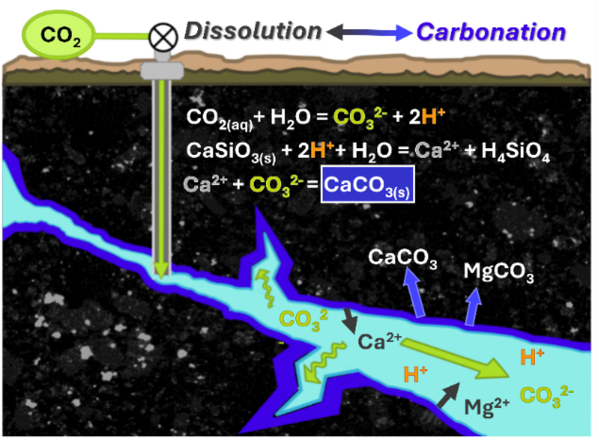
Mineralization is the most secure form of carbon sequestration, as injected CO2 is naturally converted into a solid phase, preventing unwanted migration out of the storage complex. Successful pilot projects at the CarbFix (Iceland) and Wallula (Washington state, USA) have demonstrated feasibility beyond lab scale. In the U.S., most mafic formations with sufficient thickness and reactivity to support mineralization are in the Pacific Northwest, Hawaii, and offshore regions.
To address climate change, it will be important to utilize our full range of GCS options to match CO2 capture potential, whether storing large volumes of CO2 in saline aquifers that underly much of the continental U.S. or mineralizing CO2 in regions with access to basalts.
Anne Menefee is a faculty member of the Institute of Energy and the Environment. She is also an assistant professor in the John and Willie Leone Department of Energy & Mineral Engineering. Her research is broadly focused on advancing sustainable energy and carbon management systems. Much of this work targets subsurface systems that can be engineered for low-carbon energy production and carbon sequestration, which are indispensable to avert the worst impacts of climate change.