23-minute listen/watch | 17-minute read | 1-minute teaser
Carbon dioxide (CO2) is abundant and stable, making it difficult to convert into something useful without a lot of energy. Researchers are using advanced computational modeling to find a catalyst that can efficiently transform CO2 into valuable products. This could turn CO2 from a climate issue into a valuable resource.
Transcript
Nelson Dzade
So I would say that catalytic conversion of CO2 into other fuels and valued chemicals is going to be highly beneficial to the environment, the economy, and to the global supply of energy in general.
Host
Welcome to Growing Impact, a podcast by the Institute of Energy and the Environment at Penn State. Each month, Growing Impact explores the projects of Penn State researchers who are solving some of the world's most challenging energy and environmental issues. Each project has been funded by the institute's Seed Grant Program that grows new research ideas into impactful energy and environmental solutions. I'm your host, Kevin Sliman.
Carbon dioxide is abundant in our atmosphere. It's also a stable, inert molecule. This means a CO2 molecule wants to remain a CO2 molecule. Generally, it doesn't react with other molecules under most conditions. And although it can be chemically converted, the conversion is inefficient and energy intensive. In other words, it takes more energy to convert carbon dioxide than it would ultimately yield. However, a catalyst may change that scenario. In this episode of Growing Impact, I speak with a researcher who's using computational power to identify a sustainable, economical material that could be used as a catalyst to convert carbon dioxide into useful products.
Hi, Nelson.
Nelson Dzade
Hello, Kevin.
Host
How are you?
Nelson Dzade
I'm doing great.
Host
Can you introduce yourself and provide your name, title, and a brief background on your research?
Nelson Dzade
So yeah, it's a pleasure to join you today as we discuss this important topic. So first of all, my name is Nelson Yaw Dzade. I’m an assistant professor in the Department of Energy and Mineral Engineering here in Penn State. And currently I lead a materials and mineral theory group. So basically, our idea is we specialize in the development of advanced theoretical metals to unravel structure property performance relationships in solid state materials. So basically what that means structure property performance relationships is basically materials come in a particular structure. And therefore, that structure would dictate the property they have which is inherent to them. And that property therefore will influence how they perform for the kind of application you are interested in. So understanding this relationship is something that can be very challenging.
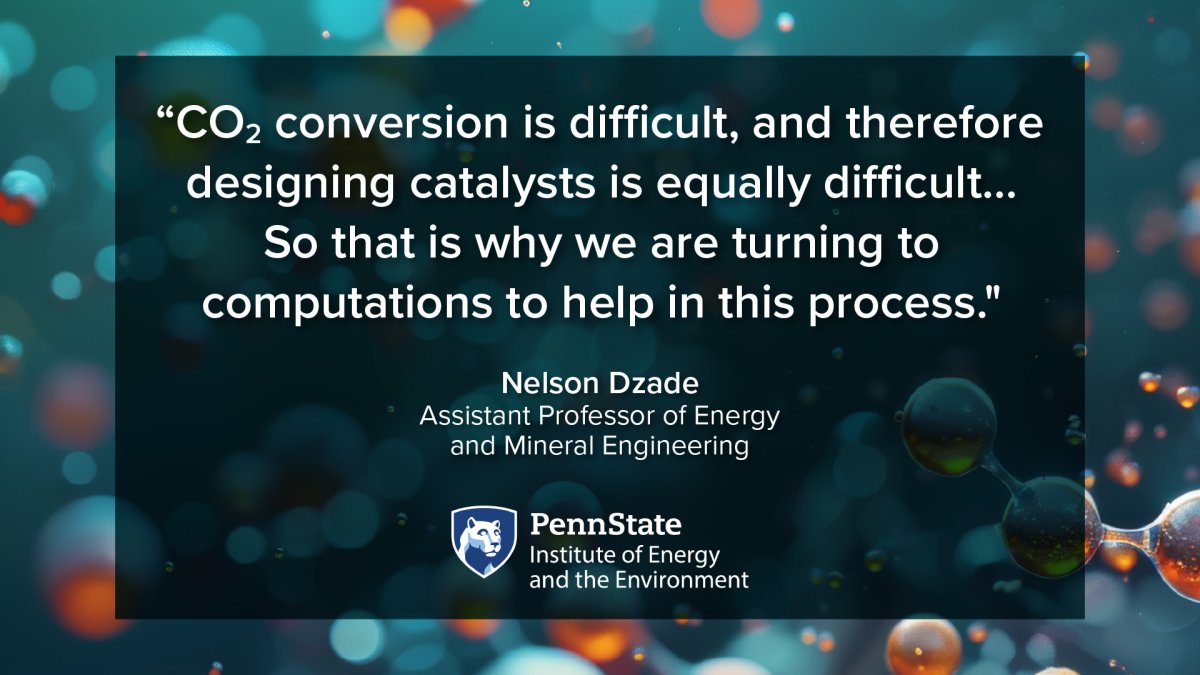
So our goal is to use advanced computation to really unravel those intricate structure property relationships. And the goal here basically is that we need materials for all kinds of things. And these materials, their performance lies at the heart of all our renewable energy technologies and devices that we are interested in. And computation is now at a stage where it can contribute to developing and new materials and understanding complex chemistries. So most of the thing we do is synergy with experiments. So we work extensively with experimental groups all across the world. And our goal here is basically to really provide them the detailed understanding and the fundamental science that they need to really rationally design and engineer the novel materials that are of interest for the different applications.
Host
The seed grant project that you're working on now, that was funded through IEE, is looking to design catalysts that can convert carbon dioxide into fuels. Is that accurate?
Nelson Dzade
That's absolutely true. Yeah.
Host
All right. So first, can you tell us a little bit about the inspiration behind the idea and then why you're working on this?
Nelson Dzade
So yeah, the project, which we call “Bio-inspired, iron sulfide and nickel catalyst for CO2 conversion,” is a project that is dear to my heart. And we all understand already why we should be doing something to mitigate or alleviate carbon dioxide accumulation in the atmosphere. And you will see that doing so is not that trivial.
So if we want to decrease the concentration of CO2 in the atmosphere, we know all of us, it’s a global concern to all of us, but it remains one of the most challenging processes in chemistry and contemporary science in general. I call it... it is a stubborn molecule. Basically, I call it’s an inert molecule. It’s highly thermodynamically stable.
Typically, the standard formation of this material would be like -394 kilojoules per mole. So it's really a fairly stable molecule. And that is why it accumulates in the atmosphere. And it's inert; it doesn't want to react with a lot of the material that we want to design to, to really bind it. So this stability of the molecules is what's actually making it very difficult for us to convert, and therefore, even if we want to really do this transformation, then it's going to require a lot of energy. That would come in the form of maybe high temperatures, high amount of electricity or photons, or even a stream of reactive reagents. So our goal therefore then is to find catalyst material that will be able, first of all, to activate this molecule, to bind a molecule, activate the molecule.
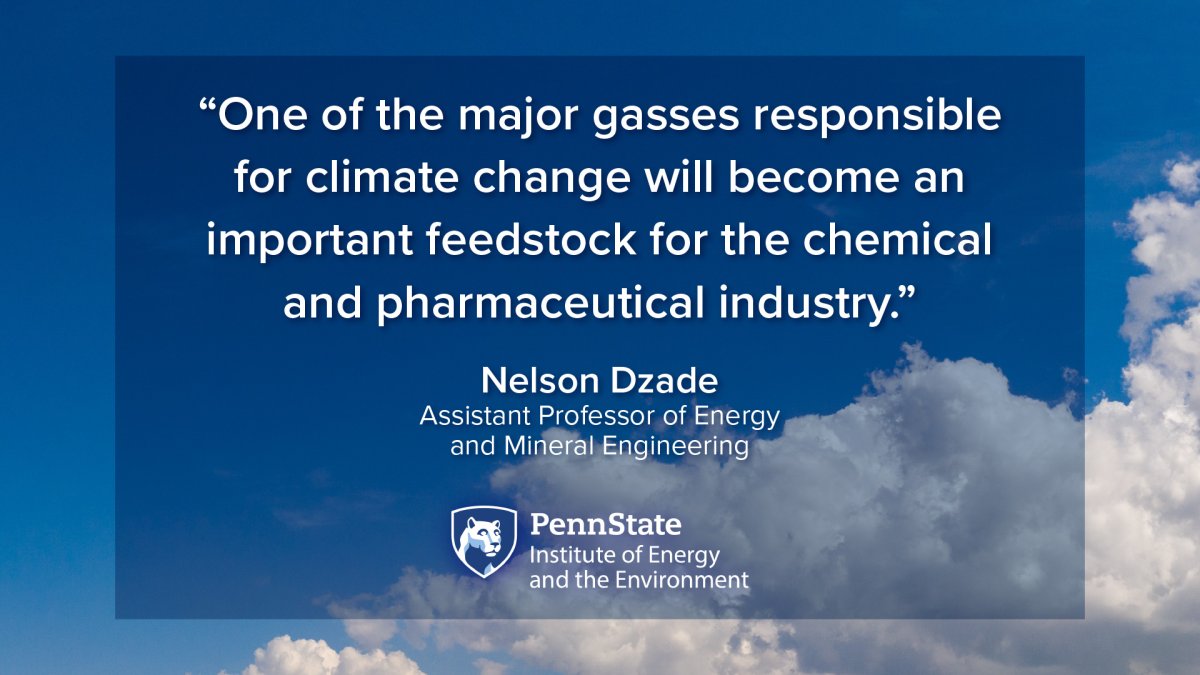
And therefore we can then convert it to something that is very useful. So we have to look around. What systems are going to do that for us? What can... what can we learn from? What can we take inspiration from? And that is why we came up with this idea of the bio-inspired catalyst, which came in the form of iron sulfide. Why iron sulfide chemistry? So the idea here is that despite the thermodynamic stability of this CO2 molecule that is very stable and inert, biological systems or enzymes are capable of both activating the molecule and converting it into a range of organic molecules under moderate conditions. For instance, if we take a biological enzyme of what we call a carbon monoxide dehydrogenase, in short CODH, this molecule can actually efficiently and reversibly convert CO2 into CO.
So and then we started to explore the chemistry of these biological enzymes. What is really the active site? The active site in catalysis simply means the site that is going to drive the reaction. So it's a gigantic biological molecule. But where is this reaction taking place? So, looking at it from the microscopic view, you will see that generally the CODH biological enzyme has its active site basically composed of iron, nickel, and sulfur in a cubane structure. So basically okay, so if this is the principle, core element driving those reactions in those biological enzymes, do we have material system that also have in these compositions of iron, nickel, and sulfur in a similar kind of structure that we can mimic, basically?
So that is what we then turn our attentions to these, what we call in the bio-inspired iron nickel sulfide minerals -- chemistry that we want to use for this type of CO2 conversion. And basically, again, not just the biological enzymes, prove that there's been a lot of theories already. Something we called, iron-sulfur world hypothesis. This was a hypothesis that was promoted by a German chemist. He has a very difficult name, but I would try to pronounce it. I think he’s called Günter Wächterhäuser, and basically the idea here is that he proposed that, even in the prebiotic chemistry, a lot of the things that happened that led to the origin of life were catalyzed by iron sulfides, and these were happening in hydrothermal vents, which are basically, a well beneath the ocean floor where magma meets the sea water and they form these gigantic chimneys, and they are all iron sulfide.
And life is thriving there, and organic molecules are formed. So he postulated that, yes, this iron sulfide should be playing a large role in prebiotic chemistry. So tying that back to the natural enzymes, there's a lot of incentive then to really take inspiration to, to really look at iron sulfide mineral in chemistry in general. Can they do this if we take them? So that's what we've been doing. And so far it's been really, really promising. We did find out, yes, a lot of the iron sulfide can actually bind the CO2 molecule, activate the molecule already and therefore give them the state we call precursors. They can become precursors. We can hydrogenate them to a wide range of other chemicals and other fuels that is of interest to us. So that is where the inspiration is coming from and why we want to look at iron-sulfide-nickel catalysts to really see how effective they will be toward CO2 conversion. And generally we, our goal here is to convert CO2 to chemicals like formic acid, carbon monoxide, methane.
We want to look at ethylene as well. And ethanol chemistries are the things that we are actually exploring. Which of the chemistry would do what or be selective towards which products and which kind of surface chemistry would drive specific type of reaction. Just to really make you understand CO2 conversion chemistry. So basically CO2 is a linear molecule, okay? So that is why it's very stable... very stable. And if it's linear and inert you can do anything with it. So basically the first step to any CO2 conversion process is to activate the molecule. So when we talk about activation, it simply means that the CO2 molecule needs to bind to a catalyst that will give it an electron. And it once it picks up an electron, it will become bent. It will no more be linear anymore. So it will form a bent geometry and then it will have a negative charge on it. So that negative charge is now hungry for protons. And that is why any hydrogen around, it's going to quickly grab it. And that's why you start to convert it.
Host
Is it always the idea that carbon dioxide is turning into a fuel, or is it just turning into a separate product? Are all the products that you're talking about, fuels of sorts, or are these just a different product outside of a fuel?
Nelson Dzade
The reason why time we talk about CO2 conversion to fuel and chemicals is because we can produce ethanol. If you produce ethanol or methanol, these are things that can be used as fuel. So CO2 itself is utilized in a wide range of things. We can use them in polymers. But the goal here is you want to convert them.
Host
Is converting CO2 to a fuel a new idea?
Nelson Dzade
I would say it's not a new idea, but the key thing is the accelerated global warming and these days, which is threatening to alter all the long-established patterns that we know -- rainfall, temperature, our ocean currents, our weather, and is what is really bringing these more into our limelight. So overall, I will say that this is something that has gained a lot of traction in the past decade where there's a massive progress in developing CO2 electrolysis technology, basically using renewable energy to convert CO2 into the fuels and the chemicals that are of interest to us.
Host
The products that you're talking about, what kind of areas could you see them being used in?
Nelson Dzade
Formic acid is the simplest of carboxylic acids that we have around, but we see them used in processing our textiles and our leather. So the leather chemistries we have, the textile that we using is all going... Formic acid is the principal, chemical, chemistry used there. So, we use formic acid also as a preservative.
Ethylene is also one of the most commercially produced substantial chemical feedstock, which is using polymers. Basically all the polymers we think about are going to be coming from ethylene chemistry. Most of our industrial chemicals are typically going to be coming from ethylene. And the beautiful thing also is that we live in the part of the world where even our fruits are not allowed to ripen on the tree before we pluck them, because they have to come from somewhere. So you realize that if they pluck fruits which is unripe, they have to make them ripe before you and I buy them from the grocery shops. So ethylene is one of the key chemicals that are used in controlling the ripening process of citrus fruits like tomato, banana. And then you see, methane generally, it's kind of a gas we can use for light and heating.
So methane is going to find itself in our homes and even in our cooking. Methane is always going to be utilized in most of those, and it’s used in the manufacture of all kinds of organic chemicals. So the last two that I mentioned is basically the, the methanol and the ethanol, which can come in the form of the fuel.
And these days, for sure, there's a lot of the world, we want to envisage for ourselves, we are looking for fuels that will help reduce the amount of CO2 we release. So ethanol and methanol generally can also be very useful in those domains. But apart from them being used as fuel, they can also find themselves in all kinds of other household products. If you take ethanol like these, you can see, things that we talk about deicer or antifreeze, are typically are going to be coming from ethanol as well. So we use it for all kinds of application. And therefore we need to produce large tons and tons of it.
Host
What are the implications of this project is successful?
Nelson Dzade
So I would say that catalytic conversion of CO2 into other fuels and valued chemicals is going to be highly beneficial to the environment, the economy, and to the global supply of energy in general. And, so basically, we can use CO2 as a building block to all the things I've just already mentioned, just products, for us. And it's therefore going to be very exciting exercise we are doing.
But one thing that is clear, which I want to state, is that it is very clear that if we are able to emulate nature -- because this process, we call it bio-inspired -- so, it is very clear that if we are able to emulate nature and successfully convert CO2 into useful chemical intermediates without the need of any extreme conditions like temperature and pressure, the benefits are going to be very enormous.
You will see that one of the major gases, which is responsible for our climate change will become an important feedstock for the chemical and pharmaceutical industry. Catalysis in general is a billion-dollar industry. And every year, it’s creating massive jobs. So it's going to benefit the economy. It's going to save our environment. Number one is going to reduce air pollution.
If we are successful because lowering the CO2 level in the atmosphere will lessen the impacts on climate change and benefit plants and animal life. Number two would be the value-added chemicals. Again, if we convert this into like ethylene and ethanol, we could provide raw material for our everyday lives, used in products in plastics, polymers, and detergents.
And the last one is the clean transportation. For sure, we need all of that. And if we can provide produce more of our ethanol, more of our methanol and, even, an intermediate product we call dimethyl ether, we can really reduce the amount of toxic particulates in our fuels.
Host
So let's talk about the catalysts. So it's this billion-dollar industry, the idea that you're utilizing nature or being inspired by nature. Can you talk a little bit about the catalyst and the materials that you're working on?
Nelson Dzade
So basically, because the molecule itself, it's such a very robust, stable molecule, we got to kind of weaken the bond. And that's why the catalyst comes into the picture. So catalysts, basically their job is to speed up the rate of the conversion process. So to speed up our rate of reaction. So the reason why we need a catalyst here basically is number one, to be able to react with our stubborn molecule, the non-reactive molecule CO2, activate the molecule, and then speed it up the conversion process for us. And if we speed up the process, it means our energy input will also come down. And that's what we really want to achieve. We don't want to pump in too much electricity into the conversion process. So the idea of developing these biological-based enzymes, it's becoming of importance because the things we want to mimic them by, are prevalent in nature.
The surface structure of these iron sulfides that we are looking as catalysts, they are structurally very similar to just the prominent active sites in these biological enzymes. So if the structure is similar to what is dominant in the natural enzyme, then the properties are going to be similar and therefore performance should be similar. That's the kind of connotation that goes with catalysis in general. So that is the reason why we strongly believe that this iron sulfide chemistry, which have very similar structures to these biological enzymes, are going to be very, very important for us to explore. Catalysis is all about electronic structure. So when we talk about electronic structure, we need to be able to give an electron to the molecule.
It has to come from somewhere, and a catalyst has to be able to provide it. So that is the idea we want to have. How can we engineer the catalyst such that it has this capacity to donate electron, give electrons to the incoming CO2 molecule and therefore bind it and then activate it and therefore convert it? What should we introduce into the matrix to make it better?
What should we introduce into the matrix to make it more stable? Because you want to use your catalyst over and over again in terms of scalability. So what should we introduce into the catalyst that is going to help the catalyst improve the stability and therefore utilize it in long term range?
Host
Can you tell us a little bit about the computational aspect of the project and how you're using computing power to make progress?
Nelson Dzade
Yeah, I think computation, where we mentioned it, particularly in catalysis, is the go-to now. It's just playing an important role in catalysis design or materials design. And the reason why that is so, is because number one, as I said, CO2 conversion is a formidable challenge for all of us. And it's not just a trivial issue, it's very, very difficult for us to design new catalysts. First of all, conversion is a difficult thing and therefore designing catalysts is equally going to be difficult. So historically, the development of catalysts has largely been dependent on trial and error. That's what we are known to do experimentally. But that approach of scientific discovery, it's long gone.
It's not prudent. It's an inefficient, costly, and wasteful approach to designing catalysts. So that is the reason why we are turning to computations now to help in this process. We want to speed up the process of the discovery, minimize waste as well as the cost. So if we want to really achieve the catalytic performance we are interested in, basically the activity, the selectivity, and the stability of the catalyst, it's going to depend on us exploiting many degrees of freedom of materials development.
For instance, we need to look at multiple chemical compositions. We need to look at nanoscale structures. We need to tailor the electronic properties. But these are things that are very difficult to do experimentally. They are not things that you can just go and do, trial and error, and mix this and this, you won't get them.
Nelson Dzade
So the ultimate goal in catalysis basically is for us to have enough knowledge about all these factors that would dictate the catalytic activity, and therefore we will be able to tailor-make the catalyst atom by atom.
Host
I noticed in your abstract it talked about students. Can you talk a little bit about why it's important to have students participating in this, and maybe what that holds for the future?
Nelson Dzade
Yeah, absolutely. I always say that students are going to be the next people to carry the torch. And we need to pass on our knowledge. And we live in an era where a lot of students are not really engaging in research, and therefore they gain all these theoretical knowledge from the classroom, but they have no research experience to really see the value of the knowledge that they have received. Catalysis is the backbone of all industrial processes, and all the chemical reactions that are going to happen are going to be driven by catalysts. So catalysts are not going away. We need people to be able to understand the chemistry behind catalysts. How do they work? Why should we design new catalysts? Understand these processes. And therefore, we as educators typically really have to think this through very carefully.
We have a responsibility basically to nurture this fascination and appreciation for the chemical science and therefore train a new generation of people who will be inspired by catalysts in general and want to work in that space. So the reason why I thought it very wise was students are going to be very useful for this project, not only for them to do the project, but beyond doing the project and getting the result out.
I really want to inspire people, basically. A lot of these young ones who came to work with us, and now they see a colleague to tell them CO2 bind and it do it, and to see the catalyst binding the CO2. It's just like, wow for them. And that brings a lot of curiosity in them, a new adventure that they want to explore. So this is really what science needs now.
Host
I like to ask this in two ways. So at the end of the seed grant, because I know that's a short period of time, but also maybe if you want to look at it further down the line, should this, should you find success in more funding down the road—how would you define success for this project?
Nelson Dzade
Success can mean a lot of things for different people. And that's why I would say the success of this project could range from different things. And number one is what we would call successful again is first of all, just using these advanced computations to sift through all these multiples of high degrees of freedom to really predict and nail down specific chemical compositions of this iron sulfide chemistry that is really going to do the CO2 activation and conversion for us. If we are able to achieve that successfully, and these materials are also made, basically we’re nearly there. They are made and they are tested, and we see them working and really doing this. That will be a fantastic success for us and something that I will be thrilled about.
And the second form of something I will call define success again, is to see that the student component we just talk about already: taking this project beyond this. A lot of them come though master’s, but they are continuing their PhD in a similar trajectory.
So at the end of the day, they are going to have a very big picture of catalysis and the chemical science industry in general. And this human development part of it, it's also a success story that we would tell that, look at this seed grant that came with this new student. And then beyond the funding, this student has been trained now got a PhD and is in the area of catalysis and therefore can become a contributor to the field.
Nelson Dzade, thank you so much for taking time. I really appreciate just being on the show and just in talking about your project, it was fascinating to learn about and it's really hopeful. Thank you so much.
Nelson Dzade
It's a pleasure, Kevin. Yes, a pleasure.
Host
This has been season five episode one of Growing Impact. Thanks again to Nelson Yaw Dzade for speaking with me about his project. To watch a video version of this episode, and to learn more about the research team, visit iee.psu.edu/podcast. Once you're there, you'll find previous episodes, transcripts, related graphics, and so much more.
Our creative director is Chris Komlenic, with graphic design and video production by Brenna Buck, marketing and social media by Tori Indivero, and web support by Jon Stabinger. Join us again next month as we continue our exploration of Penn State research and its growing impact. Thanks for listening.