18-minute listen | 14-minute read
Waste heat has been a challenge that scientists and engineers have been pondering for decades. What can be done with this lost energy and can it be harnessed in a useful way? As combustion and technology improved, the percentage of waste heat has decreased, but it is estimated that up to 50% of all industrial energy is lost through waste heat. If that heat could captured or used in a meaningful way, society would move closer to a circular economy. While Derek Hall and his team explored how different battery chemistries might change a battery’s power and energy output, they discovered new opportunities for turning waste heat into stored electrical energy.
Transcript
INTRO: Energy security and climate change continues to impose practical limits on how much energy we can consume, right? So, every time we burn something or find an energy source, any extra bit of useful work we can get out of that goes a long way.
HOST: Welcome to Growing Impact, a podcast by the Institutes of Energy and the Environment at Penn State. Growing Impact explores cutting-edge projects of researchers and scientists who are solving some of the world’s most challenging energy and environmental issues. Each project has been funded through a seed grant program that’s facilitated through IEE. I’m your host, Kevin Sliman.
Batteries have undergone numerous iterations since Alessandro Volta invented the first true battery in 1800. And those developments continue today. On this episode of Growing Impact, I speak with Derek Hall, an associate teaching professor in the College of Engineering. His team’s project, titled "Increasing Power Densities and Cycle Efficiencies of Novel, Thermally Charged Flow Batteries Using Advanced Flow Cell Topologies," started by exploring how different battery chemistries might change a battery’s power and energy output. During this investigation, the team discovered new opportunities for turning waste heat into stored electrical energy. The discovery led to a patent, publications, and a Department of Energy project for the agency’s Energy Storage Grand Challenge.
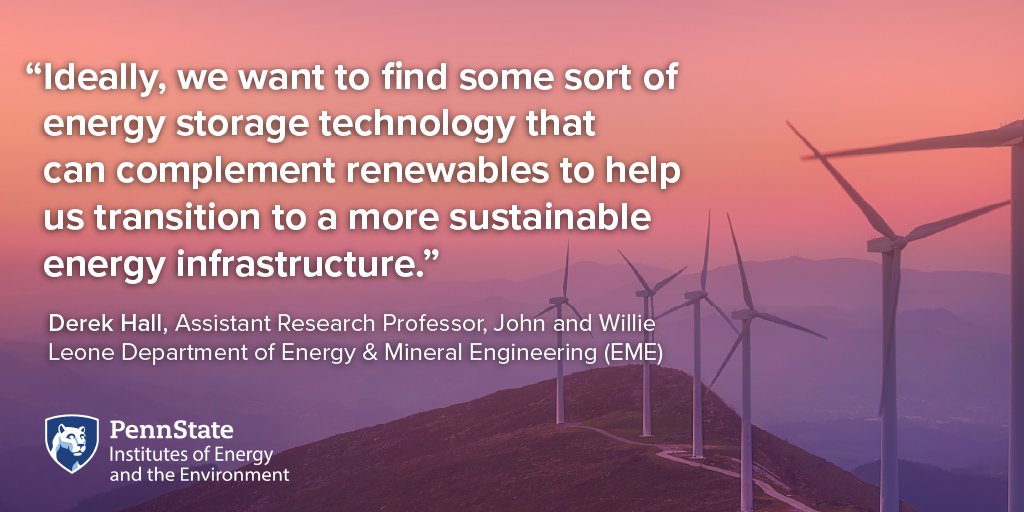
HOST: Derek, welcome to Growing Impact.
Derek Hall (DH): Hi Kevin. Thanks for having me.
HOST: Could you give me your title and your research focus?
DH: My name is Derek Hall. I'm associate teaching professor in the Department of Energy and Mineral Engineering. I'm also the program chair of the energy engineering undergraduate program in our department. My research interests are electrochemical systems. So I focus on electrochemical energy storage, electrochemical energy conversion, and sensors. And generally anytime electricity and chemistry get together, I usually find something to be interested in.
HOST: Stored electrochemical energy—Is that synonymous for battery or is that different?
DH: So stored electrochemical energy is synonymous with battery. I think there are a few other things that do store energy electrochemically that aren't batteries. A few examples are the technology that are used in the hydrogen economy. So if you're using a water electrolysis, for example, you'd be storing the energy in hydrogen. So it's sort of like chemical energy, except the method that you put it in and pull it out is through an electrochemical device. But I think for most, almost all like normal applications, it would always be a battery.
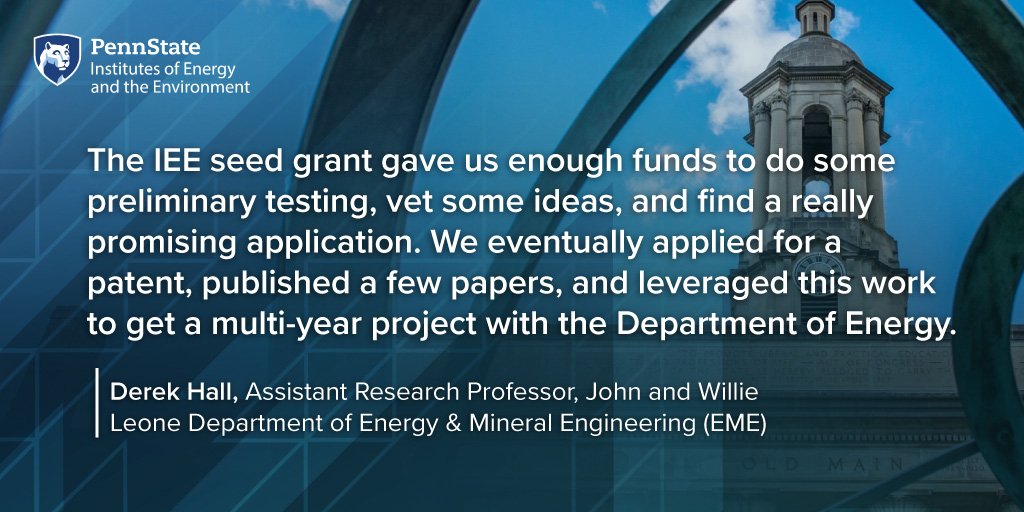
HOST: So this all started well, it probably didn't all start, but at least part of it was connection to a seed grant that came through the Institutes of Energy and the Environment. Can you provide some background on the project and explain what you were aiming to do?
DH: Our team was looking at how electrolyte compositions affect electrochemical reactions, and how we can tune electrochemical reactions that do things that we wouldn't otherwise expect from them. The system that we were working on related to copper—the copper redox potential and the kinetics of that reaction are well known in some environments. And what we wanted to do is essentially change that environment, see if we can modify those parameters and what would come out of that. So some of the basic questions we look to do is if we change what's in the electrolyte and how much of it's there, can we change the power and energy output of an electrochemical system? And are there any obvious limits to those connections? And from that, we found out that there are some promising combinations that we could do for harnessing low-grade waste heat to convert it into electrical power using that. So we didn't really go into it with that as the final outcome. But that's just what we found along the way.
HOST: Interesting. So the waste heat was it was a discovery that you weren't necessarily going in and looking for, but happened to be something that came out of it.
DH: There are some methods that use low-grade waste heat to convert it into electro-chemical power, but that wasn't really what we were using the seed grant for to start with. We’d use some of them inspired by some of it to what we chose. But we were thinking of flow batteries or all sorts of other technologies. And we ended up finding that the particular chemistry we were focused on ended up being much better for this low-grade waste heat application. So yeah, very exploratory to start with. We're not really sure where we're going to come out with.
HOST: In the abstract, your team sites that about 20 to 50% of all industrial energy is lost through waste heat. Is this idea of waste heat new? A new concept, I guess. And have people been thinking about this for a long time? I guess let's start there. Is it like, oh my gosh, we're losing, we're burning coal or whatever it is and we're losing all of this energy through heat. Is this a new concept or have we been doing this for centuries? And people have been trying to tackle this waste heat issue?
DH: Yeah, it's the latter. Engineers have been struggling with this for centuries, and we're always getting better. The original combustion systems we used, they were much worse, right? So they've been working on this for a couple of different levels. The turbines for the furnace, they're always trying to maximize their efficiency, but there's some inherent limits. So they've even looked at other systems like district heating and other complementary devices they can put it into always tried to make the number of larger. But even after all these efforts, we're still huge percentages of the energy that we use is being lost. So there are a number of devices that people have looked into to see, well, how much lower can we derive the temperature before we just can't use it anymore?
HOST: Is that part of the inspiration? Is that what made the team think about waste heat? I mean, you gave a little bit of connection into that it wasn't necessarily what you were going and looking for, but you found out that that was actually one of the benefits from some of the experiments in the project that you were doing?
DH: Yeah. So their collaboration on the seed grant, some of our collaborators, they had, they develop devices that did this in the past. So they had some awareness of this space. And I think once we started discovering some things, they found that there were good applications over here. What really caught our interests was that when this emerging area of these thermally regenerative batteries, which is what we call this particular device. There was a lot of overlap with their desirable outcomes in the design with that of a flow batteries, which is things that we've worked in in the past. So once that connection became clear, that what we knew in some of these previous projects that we've worked on had a good overlap with this, that's what really got us excited saying we can use this knowledge from this other field. And what we've learned here to try to make this low-grade waste heat to electrical power area better.
HOST: Maybe a quick definition here. So you've used the term flow battery and thermally regenerative battery. Can you give me just a layman's overview of those two things?
What is a flow battery?
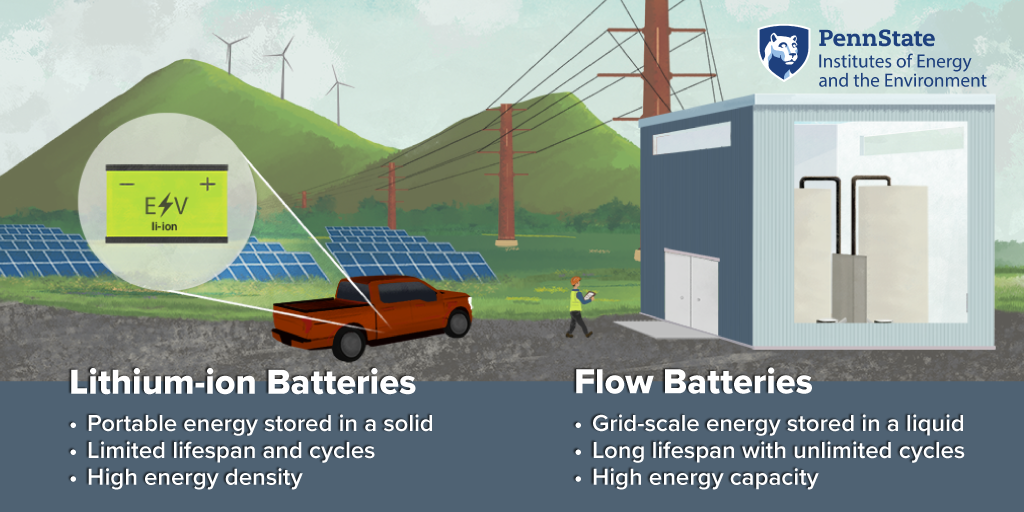
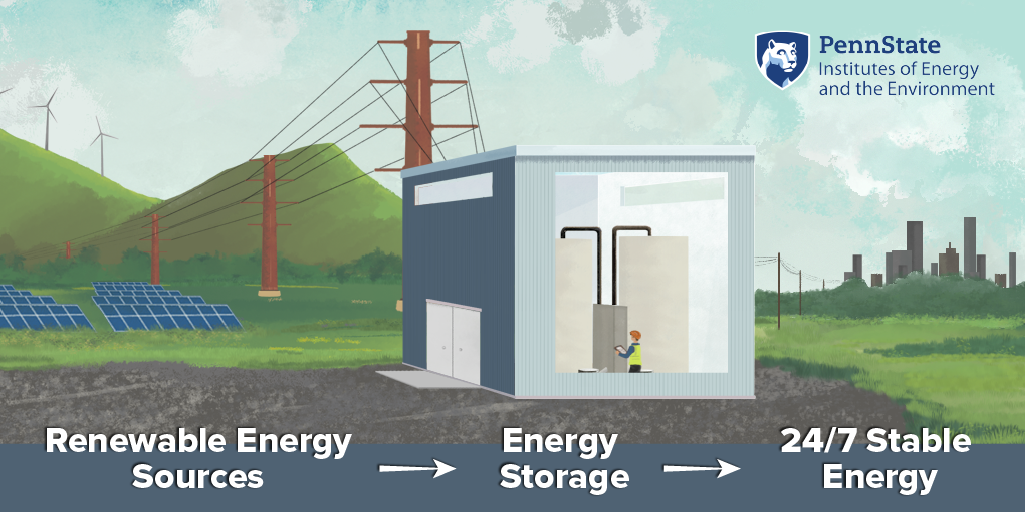
DH: A thermally regenerative battery, instead of putting electrical energy in, we actually put heat in, which is another form of energy. And then we convert that into electrical power. So it comes in as thermal energy, gets stored as chemical energy and then comes out as electrical energy. So different charging mechanism but similar idea, put energy in stored over time and then withdrawal when you want it. Flow batteries are actually, it's an old energy storage solution was invented by NASA back in the '70s. These batteries, unlike the normal ones you would have in your laptop or your phone, they actually store the energy in a liquid. Normally it's ends up being stored in the solid in a typical battery. You charge and discharge them through a power stack. So the storage of the energy in these liquids, which are usually in large tanks, are separate from the charging and discharging station, which is usually the stack, looks like a bunch of library books. And so the liquids are usually water and they contain metal ions and other salts in them. And they are pumped into this stack when they're needed, but then they're brought back. So back when they were invented in the '70s, there wasn't really an energy storage problem that they worked well for mostly because water is extremely heavy. So if you wanted to store energy, it has to be something that's not moving, which basically rules out the old Walkmans, batteries, laptops, phones, cars. So everything that we want energy storage in. So until renewable energy sort of came on the horizon, and we can produce lots of electrical energy, but not necessarily when we want it. The first, I would say first large-scale start, the start of a large-scale application for grid-scale energy storage or storage where you can build it the size of a building. You don't have to move the building, but there's still a use for it. These flow batteries have some advantages in that space but may not work for other things like rocket ships, which is why they sort of like a solution without a problem basically. Now we have a problem it works for. So a lot of people have been digging back into it to see, can it solve some of these new problems that we have. Thermally regenerative batteries (TRAB) that were first proposed in this area—so the batteries that can be recharged with heat—they followed the same sort of design trends that lithium ion or the classic double a batteries would. So there's a metal or some solid that's part of the energy storage process. And a lot of these older ones in the TRAB space would use this. So our design was one of the first to take this flow battery concept and apply it to a thermally regenerative battery. So like a flow battery are the electro active species are the things doing the energy storage. They're all contained in the solution. So we have our power stack, we have our tanks. And you were essentially copying that flow battery design in making a thermally regenerative battery with it. But to do that, we needed a chemistry that would allow for that. So that was that was what we found through the seed grant, was we found this, we call an all aqueous, thermally regenerative battery, but that essentially just means everything's in the liquid. The active species.
HOST: Let's talk about challenges or obstacles and converting heat directly into stored electrochemical energy. What are you seeing in your area? Are there things in the lab that are challenging just chemically or scientifically, or they're outside influences like regulations or anything like that, economics? I guess where are you seeing issues and how do they impact that work?
DH: That's a good question. So a lot of the devices proposed to convert low grade heat into electrical power, they all suffer from similar problems. Because the temperature is very low, the available energy and the temperature difference is typically not very large relative to the environment. And what that does is it limits the energy efficiency and the power density—which is essentially how much power you can get out of per unit volume of a device—have been quite low across the board. So the technology that we're working on is one of many in this space. And there has been quite a bit of reviews and scientific papers that discuss these metrics. You basically want to produce a lot of power, have the best energy efficiency possible at an affordable cost. And so those are the main challenges, most of which has been getting a high-power density. So some of the earlier works in this space, that power output was orders of magnitude under load via typical battery. And so what that means essentially is if you're going to build a battery or one of these in real life you're going to need that much more space in order to accomplish that. So a lot of what our work has been doing, a lot of what our work is focused on now is trying to figure out how can we maximize how much power we get so we can make these smaller and closer to this scale that would be useful for us. Those two are two big issues that has been a challenge. And then the last one is the cost. But they're somewhat related. So any solution we have to increasing power output, we need to make sure that we're not relying on materials or some process that affects the cost in some dramatic way. One example of this could be membrane choice. So there are a lot of membranes, materials that get used in batteries. Some of them are more expensive or less expensive. So if we do get a power boost from using one, if that comes at a cost, there may be some trade-offs there saying well, and get more power, but the additional cost for that benefit isn't really there. So we need to work with a lot of experts in different areas to try to find an optimal solution. It may work for one of our problems, but that may bring up new ones is somewhere else down the line. Because it's new. There's still quite a bit of unknowns, right? Generally, those are the goals, more power, less cost.
HOST: So you started this perfectly. Your team cites that the project is inherently interdisciplinary. Can you talk a little bit more about why you need experts from so many fields and such from different fields to contribute to the project?
DH: I think that's just an inherent issue with all electrochemical systems, especially like the one we're discussing today. So the phenomenon that makes an electric chemical system work are best understood by multiple engineering disciplines. So some examples are like in our flow batteries, fluid is flowing. So mechanical engineers typically understand fluid flow and porous media best. There's also heat being transferred, there's chemical reactions taking place. And essentially all of those processes need to work together to make a high-performance electrical circuit. So, I mean, if you think about the environmental implications is easily includes every department in the College of Engineering. And then especially when you're considering the cost, then you need an economist. The list of people that would be helpful to have on your team quickly grows. If it's in an interdisciplinary system like this, It's tends to be one person isn’t usually bold enough to jump in. You have to come in as a team to make sure that you're doing all the other parts justice.
HOST: And do you find yourself learning ridiculous amounts of information from those other individuals, through those collaborations?
DH: I think that's one of the strong benefits of working with people in different disciplines. I mean, you'll never know enough to be better at it than they are, but you start to understand the language, and you'll make less obvious mistakes. And that's one of the best parts about being an academic, right, that learning never stops. So the more you work with people outside of your field, the easier it is to learn something, I should say, the chances of me learning something increases a lot.
HOST: How could this project impact the world, and what benefits will come from that impact?
DH: The easy part about energy and trying to think of the impact of our energy system is you can look at the major issues we're dealing with today. So if any of this is successful or any of my other colleagues are successful. I mean, energy security and climate change continue to impose practical limits on how much energy we can consume, right? So every time we burn something or find energy, an energy source, any extra bit of useful work we can get out of that goes a long way. So this low-grade waste heat issue that we're talking about now, if we're still making glass, that typically takes high temperature, high temperature to achieve that, then at some point, you're not going to have anything left to do with that heat. If you can make a little extra electrical power out of that, that's going to stave off how much more electrical power you're going to need from something else. So the more efficient we are, the closer we get to the circular economy. So if this is successful, it brings us just that little bit closer. It’s inevitable there’ll be some sources of heat. And if we can find a way to get a little extra energy out of it, that's useful to us, that's going to have a huge impact, right? Like 20% of all of it's wasted if we even shave off 1% of that for something useful, that could add up to a really big number.
HOST: Since the original seed grant in 2019, I've been reading things that have been happening with your group. Could you give me a little bit of some of the updates about the seed grant and maybe where the project has been and then even maybe where it's going?
DH: So we were really lucky with the seed grant. It gave us enough funds to do some preliminary testing, vet some ideas, and find a really promising application for it. So shortly after the seed grant was over, we applied for a patent application for the underlying battery chemistry because it was new and hadn't been done before. We published a few papers on it. And some of that was leveraged to get a multi-year project with the Department of Energy as part of their Energy Storage Grand Challenge. So the new project that we're working on now is we have some collaborators in the industry space, some from power plant assets to kind of give us some advice about what waste heat, how much is being produced and where it's being produced. If we want to sell them a thermally regenerative battery, what sort of properties are they interested in? We're also in discussion with energy storage device manufacturers as well to kind of give us some advice about what they would look for in a promising in an output. I mean, granted the technology readiness level or the state of maturity of this technology is very, very early still. But having their input now has been really helpful to figure out what should we be aiming for in the long run. So the new project that we have, we're mostly focused on what design choices make the most sense. I talked a little bit about membranes. We're looking at different membranes, looking at electrolyte compositions, how this impacts cost and performance, and just identify any big trade-offs or big technical gaps that we see given where we are with this new chemistry so that we can better direct future efforts in this space. Hopefully someone comes along, does a better job than what we did. So we're kind of trying to paint that area and say, these are where we think the big hurdles are for the next group.
HOST: Thank you, Derek, for spending time on Growing Impact and talking about your research.
DH: It was great being here. Pleasure talking to you, Kevin. Thanks so much for the invite.
HOST: This has been season 3, episode 7 of Growing Impact. To learn more about IEE and to hear previous episodes, read podcast transcripts, and see related graphics from Growing Impact episodes, please visit iee.psu.edu/podcast. Thank you for listening.