The universe is largely at a very cold temperature, about 3 K (kelvin) or -454 degrees Fahrenheit (F). Through thermal radiation—one of three fundamental mechanisms to transfer heat (the other two are conduction and convection)—heat flows from a hotter object to a colder object in the form of electromagnetic radiation. Radiation is the only method of heat transfer that doesn't need a material present. It uses electromagnetic waves, which can travel through empty space, like the vast distance between the sun and Earth.
Keeping the sun as an example, the sun’s surface temperature is about 6,000 K. It radiates heat (sunlight) to the surface of Earth at about 300 K (or 80 degrees F). Likewise, the object on the surface of Earth at ~300 K will radiate heat to the universe. Therefore, by using the universe as a heat sink, radiative cooling provides a way to cool objects on Earth to below the ambient air temperature without any electricity input. To do this, the object must be facing the sky.
By providing such electricity-free passive cooling, the coldness of the universe has emerged as a new renewable thermodynamic resource for cooling buildings, vehicles, outdoor equipment, and human bodies. Additionally, there is the opportunity for direct electricity generation, for example, by exploiting the temperature gradient created by radiative cooling using a thermoelectric energy generator.
A quick lesson on electromagnetic radiation
Electromagnetic radiation comes in different wavelengths. As an example, visible sunlight is comprised of light in different colors, such as purple, blue, green, yellow, and red, which can be revealed by passing sunlight through a prism. Each color represents a different wavelength. Similarly, thermal radiation from an object on Earth also comes at different wavelengths, mostly in the mid-infrared wavelengths, which are between 3 to 25 micrometers. Earth’s atmosphere is mostly transparent at wavelengths between 8 to 13 micrometers, allowing infrared heat radiation from the surface to escape into space. Therefore, to achieve radiative cooling, the sky-facing object must be able to emit thermal radiation at wavelengths between 8 to 13 micrometers so that heat can be sent into the cold of outer space. To ensure that an object emits thermal radiation in the necessary wavelength range, we can use a photonic approach—an expertise of our research group—to design the emissivity of an object at mid-infrared wavelength. This quantifies the object’s capability to emit thermal radiation by engineering its materials and microstructures.

Radiative cooling
Nocturnal radiative cooling has been observed for a long time. For example, when there is a clear night sky, even when the ambient air temperature is above the freezing point of water, it is possible to see frost on grass in early morning. This is because objects can radiatively cool to below the ambient temperature by sending heat to the sky at night.
However, the peak of cooling demand happens during the day, and achieving radiative cooling under peak sunlight would be far more impactful. The major challenge is creating an object that has negligible sunlight absorption.
A transparent solution
An object under the sun typically increases in temperature. To achieve radiative cooling under the sun, the object must absorb negligible sunlight. Existing radiative coolers achieve this by reflecting almost all the sunlight, usually >96%. However, this wastes sunlight—a key renewable resource.
Our research group takes a different approach. We utilize visibly transparent material to achieve radiative cooling. This transparent radiative cooling material can emit thermal radiation while absorbing a negligible amount of sunlight (<0.9%). Concurrently, the material allows sunlight to pass through it to drive photovoltaic cells. We also use an infrared-opaque spacer to block the radiative heat transfer from the solar cell to the cooler. As the transparent radiative cooler has little solar absorption, it is insensitive to solar irradiance, and cooling performance is maintained even under peak sunlight, which the sun supplies at about 1,000 watts per square meter.
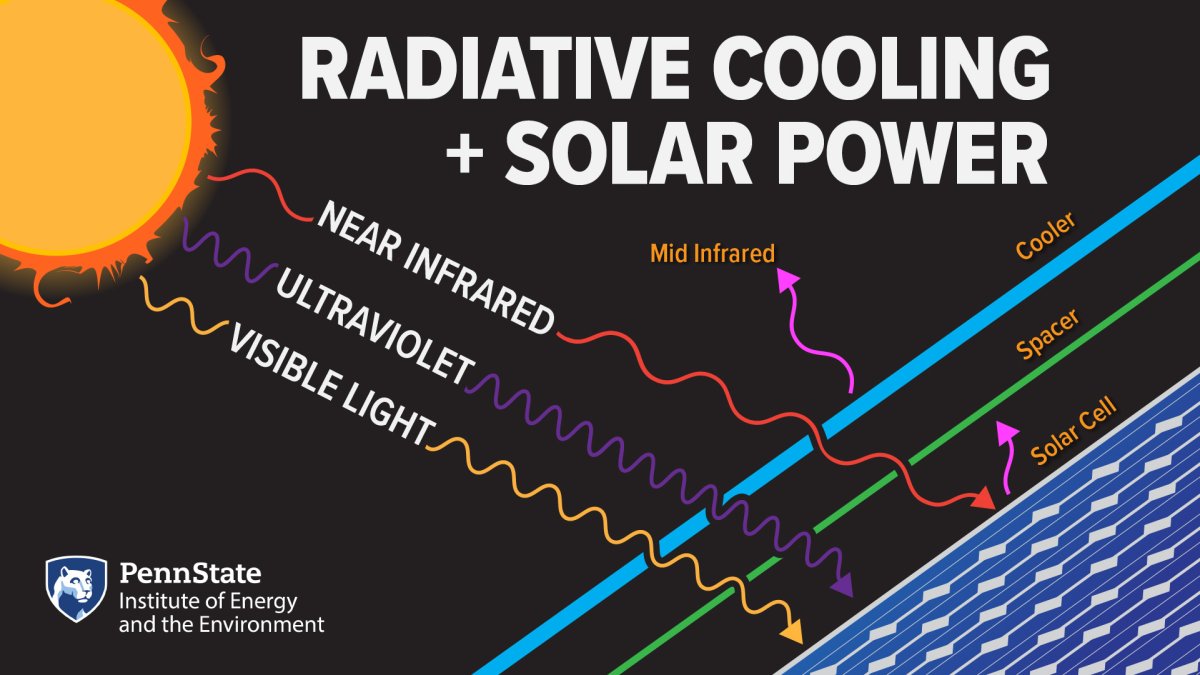
Improving upon previous attempts
There has been substantial interest in achieving simultaneous cooling and solar energy harvesting using the same space. In two prior studies, people have achieved simultaneous cooling and solar heating, where heating is achieved at 20–30 degrees Celsius (C) above the ambient temperature. Such heating can be useful for providing heating. However, converting such heat to electricity will have low power conversion efficiency due to the low-temperature differential, which is subject to a thermodynamic principle known as the Carnot limit.
In our work, we combine a transparent radiative cooler with a photovoltaic cell to achieve simultaneous cooling (~5 degrees C or 9 degrees F) and significant photovoltaic electricity generation of 159 watts per meter squared. For comparison, today’s solar panels typically generate about 200 watts per meter squared under real-world conditions. There is room to advance photovoltaic electricity generation by improving the visible transmittance of the radiative cooling materials through the use of an anti-reflection coating.
Real-world application
In our proof-of-concept demonstration, we showed simultaneous sub-ambient radiative cooling on a transparent cooler and significant photovoltaic power generation. Two potential approaches to using the produced cooling include cooling either air or liquid and then pumping the cooled material to integrate with the application's thermal systems.
Future development can include integrating cooling with a thermal system through cooling air or liquid. The dual-harvesting system could offer opportunities for energy savings in buildings, electric vehicles, and cold chain supply.
Linxiao Zhu is a faculty member in the Institute of Energy and the Environment and an assistant professor in the Department of Electrical Engineering. His research involves understanding the fundamentals of how light and heat can be controlled using nanostructured materials, as well as using the knowledge to advance energy efficiency and develop clean energy. His work includes controlling thermal radiation by taking advantage of the strong light-material interaction at the nanoscale. By using nanostructures with feature sizes comparable to the wavelength of this light, various properties of heat and light, such as spectrum, direction, intensity and many others, can be designed and tailored, leading to great opportunities for energy and thermal applications.